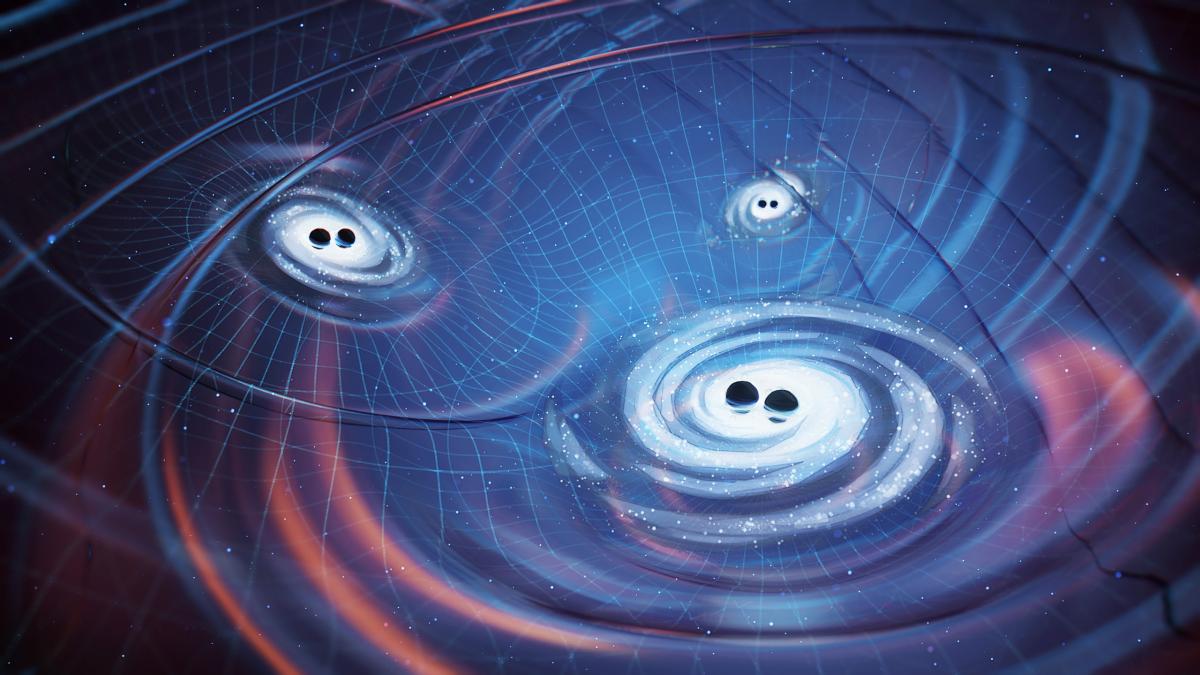
Supermassive binary black hole binary systems at the cores of galaxies produce ripples in spacetime called gravitational waves. Gravitational waves from all of the supermassive black hole binaries in the universe combine to form a stochastic background which we can detect using pulsar timing. Illustration: Olena Shmahalo
Supermassive black holes (SMBHs) lie at the centers of massive galaxies, with masses sometimes billions of times greater than our Sun, and as large as our own Solar System. Galaxies grow through successive mergers, providing the opportunity for two of these behemoths to find each other and form an SMBH binary. For decades, such binaries have been hypothesized to exist, but not a single example has been confirmed. For just as long, theorists have been debating whether or not such monstrous systems could actually form—can two SMBHs find each other after the violence of galaxy merger? If so, what physical mechanisms could bring them so close together that they are able to produce detectable gravitational waves (GWs)?
In this study, we produce simulations of SMBH binary populations that contain billions of sources, and compare their predicted GW signatures with NANOGrav’s most recent observations. We find that a number of different models are accurately able to reproduce the observed 15yr GW spectrum (see figure). While we can’t yet pin down the source of the NANOGrav signal uniquely, the measurements are consistent with expectations for SMBH binaries.
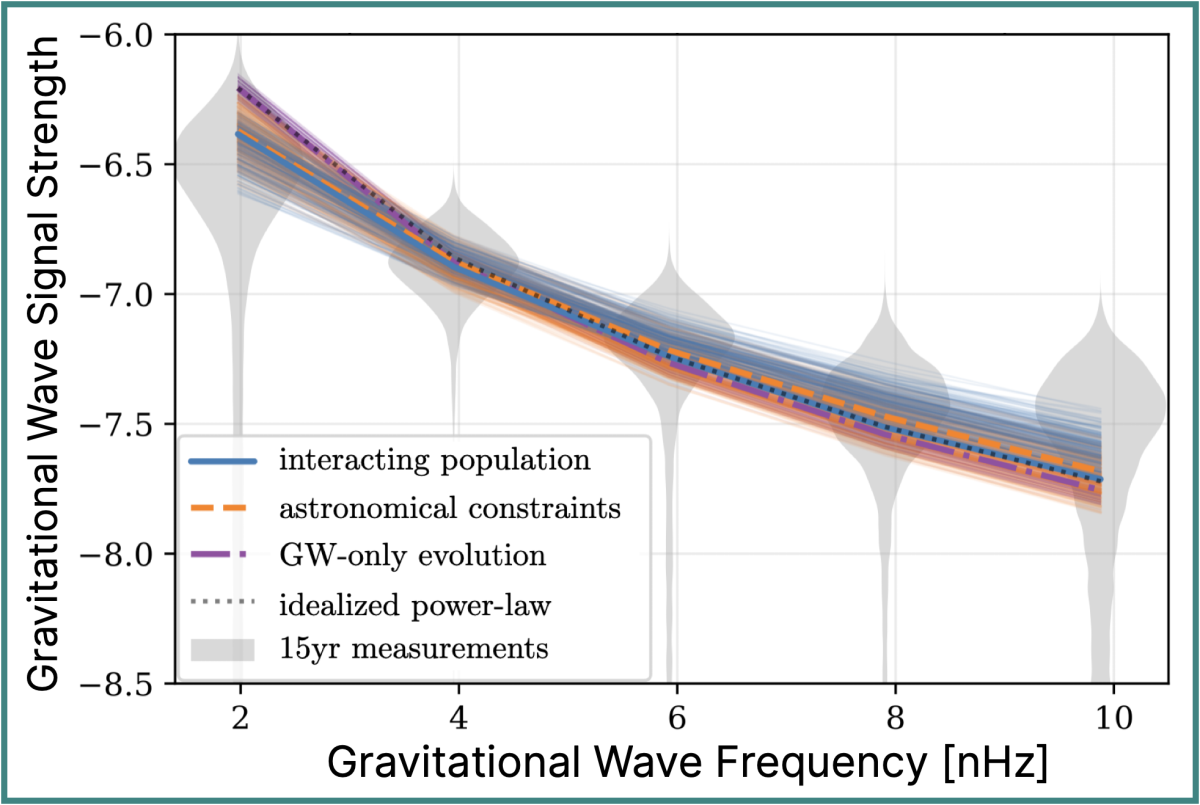
Simulated populations of supermassive black hole binaries accurately reproduce the signal detected by NANOGrav. We compare the observed characteristics of the gravitational-wave (GW) background detected by NANOGrav, shown as grey “violins,” with the best-fitting theoretical GW spectra from SMBH binary population models (colored lines). We show three different types of simulated populations, all of which are able to accurately match the data. The bestfitting populations (blue) require more than just gravitational waves: the binaries must be interacting with their host galaxies at higher frequencies than typically expected.
In our simulations, we find that information about SMBHs is encoded in the precise shape of the GW spectrum. If the signal is produced by binaries, we are already able to place constraints on their properties, such as their typical masses and distances. Based on current measurements, the amplitude of the signal suggests that SMBHs may be more common or more massive than previously thought.
Excitingly, the amplitude of the signal in the lowest frequency bin provides a tantalizing hint that SMBH binaries may not evolve in isolation—they may require interactions with the stars and gas of their host galaxies to produce the signal that we’ve detected.
Based on current measurements, the ... signal suggests that SMBHs may be more common or more massive than previously thought.
NANOGrav’s sensitivity will continue to improve as observations continue, allowing us to improve our constraints. We will also seek out variations in the GW amplitude at different positions on the sky, which could be a hallmark of SMBH binaries as opposed to alternative sources that have been proposed. Eventually we will be sensitive enough to pick out individual binaries from the background. These sources would then represent a golden opportunity for observation with not only GWs, but also traditional electromagnetic surveys, allowing for unprecedented measurements with both light and gravity.
Agazie et al., 2023, The NANOGrav 15-year Data Set: Constraints on Supermassive Black Hole Binaries from the Gravitational Wave Background. https://arxiv.org/abs/2306.16220
![]() |
Dr. Luke Zoltan KelleyLead for the Astrophysics Interpretation Paper
|