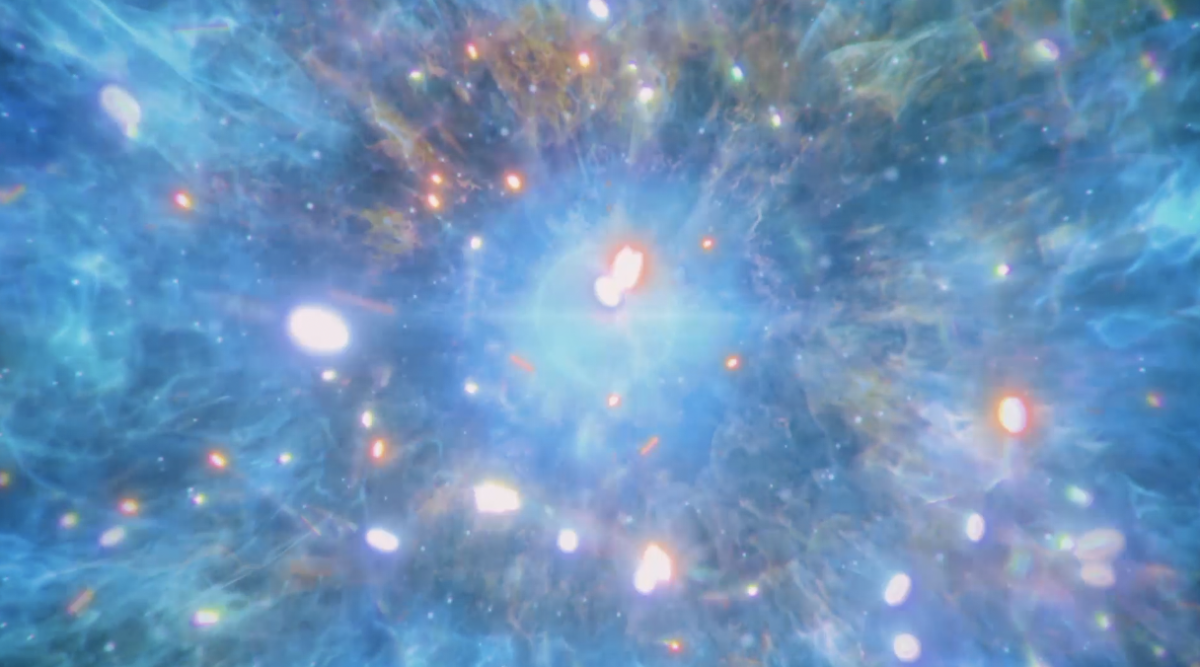
Artistic rendering of the early Universe. Credit: Little Shadow Ltd.
Our current understanding of the subatomic world is based on the Standard Model of particle physics, whose last missing particle, the Higgs boson, was triumphantly discovered in 2012. However, while being incredibly successful in describing all known subatomic particles, the Standard Model still fails to explain several key properties of our Universe: it cannot explain the origin of the observed asymmetry between matter and antimatter, it does not contain a good particle candidate for dark matter, and it fails to describe the properties of dark energy — three observations that point to the existence of new physics beyond the Standard Model (BSM). Therefore, after the development of the Standard Model in the 20th century, 21st-century particle physics now faces the challenge of venturing into BSM territory and identify the correct successor to the Standard Model.
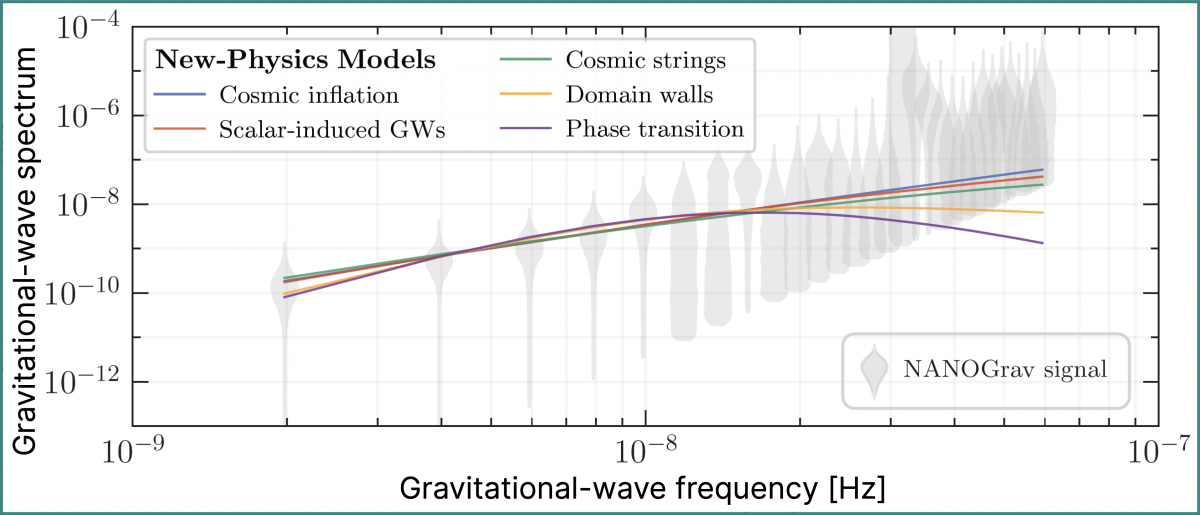
The NANOGrav signal might also contain a contribution from gravitational waves (GWs) produced in the early Universe right after the Big Bang. Here we compare the GW spectrum of potential primordial signals with the strength of the observed signal (gray contours). Each model ties to an extension of the Standard Model of particle physics; cosmic inflation (blue - exponential expansion of the Universe), scalar-induced GWs (red - large density fluctuations in the primordial plasma), cosmological phase transitions (purple - changes in the vacuum state of the Universe, similar to the phase transition from liquid water to steam). Cosmic strings and domain walls (green and orange lines, respectively) are cosmic defects, like cracks in an ice cube that occur as water freezes.
Over the years, theorists have proposed countless BSM models, many of which are currently being tested in high-energy laboratories around the world, notably at particle accelerators such as CERN's Large Hadron Collider (LHC). Current efforts in particle physics focus on several frontiers that are characterized by, for instance, ever higher energies (the “energy frontier”) or ever larger statistics (the “intensity frontier”). A new emerging frontier in this context is the gravitational-wave frontier, which capitalizes on the fact that many BSM models also predict the generation of gravitational waves in the early Universe. In the present paper, we open a new chapter in the exploration of this frontier and establish the search for new physics at what we call the “PTA frontier.”
"Such a GWB should be thought of as the gravitational analog of the cosmic microwave background … generated at even earlier times, much closer to the Big Bang."
In our work, we consider a suite of BSM models that predict the generation of a gravitational-wave background (GWB) only fractions of a second after the Big Bang. Our goal is to assess to what degree this primordial GWB, which has propagated more or less freely through the Universe since its production, could explain the signal observed in the NANOGrav 15-year data set. Such a GWB should be thought of as the gravitational analog of the well-known cosmic microwave background (CMB), which denotes the relic electromagnetic radiation produced when the Universe was as young as 380,000 years. A crucial difference from the CMB, however, is that a cosmological GWB is expected to be generated at even earlier times, much closer to the Big Bang. Detecting a primordial GWB would therefore provide a direct glimpse of the dynamics of the Universe at times that are not accessible by other means, and probe particle interactions at energies that cannot be attained in laboratory experiments. In this way, our search for primordial gravitational waves in the NANOGrav 15-year data allows us to test ideas such as grand unification (the unification of all subatomic forces in one common super force at extremely high energies) or even string theory, a proposal for a consistent theory of quantum gravity.
Many of the models that we consider can fit the data equally well or even better than the expected signal from supermassive black holes. However, there are still large uncertainties related to the modeling of the supermassive black holes signal, and more work is needed before we can conclusively establish whether the NANOGrav signal is of cosmological or astrophysical origin.
Afzal et al., 2023, The NANOGrav 15-year Data Set: Search for Signals from New Physics. https://doi.org/10.3847/2041-8213/acdc91
Corresponding Authors
![]() |
Dr. Andrea MitridateCo-Lead for Signals from New Physics Paper
|
![]() |
Dr. Kai SchmitzCo-Lead for Signals from New Physics Paper
|