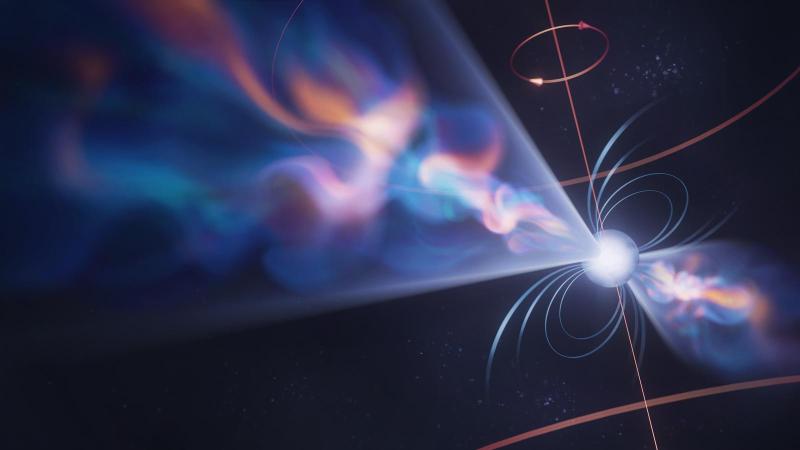
Energetic particles are accelerated along a pulsar's open magnetic field lines, producing radio emission. As the pulsar rotates, our radio telescopes detect this beamed emission as precisely periodic radio pulses. Illustration: Olena Shmahalo
Energetic particles are accelerated along a pulsar's open magnetic field lines, producing radio emission. As the pulsar rotates, our radio telescopes detect this beamed emission as precisely periodic radio pulses. Illustration: Olena Shmahalo
Stars like our Sun support themselves against their very strong gravity through energy generated from the conversion of Hydrogen to Helium in their dense, very hot cores. When stars run out of Hydrogen in their cores, they can then start to convert heavier and heavier elements. The Sun will end its life with a carbon and oxygen core, but stars that are more massive than the Sun will produce even heavier elements through this process. In fact, stars with masses roughly 10 times that of the Sun will end their lives with an Iron core! This Iron core will not be able to undergo nuclear fusion and gravity will therefore win, causing the core to collapse in on itself. The outer layers will bounce off the core in an energetic supernova explosion.
The remnants of stars with masses greater than 20 times the mass of the Sun will become black holes, but stellar remnants with masses between 10 and 20 times the mass of the Sun will become a special kind of star called a neutron star. Neutron stars are extremely dense - a teaspoon of neutron star material on Earth would weigh as much as the entire human race! They also have extremely large magnetic fields, strong enough to erase every credit card and computer hard drive on Earth...even if the pulsar was as far away as the Moon! Pulsars are a special class of neutron star which rotate rapidly and produce beamed radio emission.
Любите крупные выигрыши? Розыгрыши с миллионными выигрышами и автомобилями бизнес-класса ждут вас на официальном сайте Вавада уже сейчас! Регистрация займет пару минут, а верификация не требуется — ловите первые заносы и сразу выводите на свой счет анонимно
Courtesy of kurzgesagt.org